We discuss the magnetic force between parallel conductors. From its fundamental principles to its practical applications in preventing sag in overhead power lines.
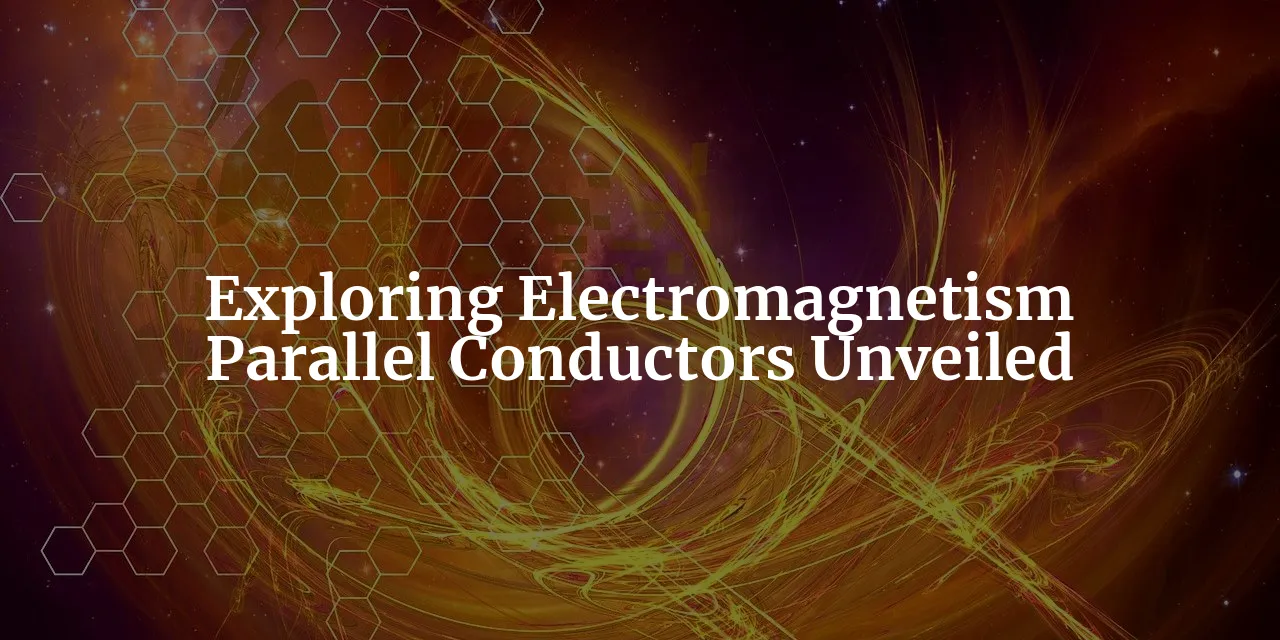
Motivation
Understanding the magnetic force between two parallel conductors is not just an academic exercise; it is a fundamental concept in electromagnetism, a cornerstone of physics with vast practical applications. This knowledge is crucial for designing electrical circuits, power lines, and even in the development of magnetic levitation systems. It beautifully illustrates the direct relationship between electricity and magnetism, showcasing how electric currents can produce magnetic fields, a discovery that transformed the way we generate, manipulate, and utilize energy.
Grasping this concept is essential for understanding the principles behind the operation of many electrical devices and systems, from simple motors to complex transformers. Beyond its scientific importance, this problem connects to broader topics like energy transmission and the principles of electromagnetic compatibility, which are crucial for modern technology and infrastructure. The ability to calculate and understand the forces at play between currents is a stepping stone towards innovation in electrical engineering and physics.
Problem Description
Consider two long, straight, parallel conductors placed at a distance \(d\) apart in vacuum. Each conductor carries a current, \(I_1\) and \(I_2\) respectively, flowing in the same direction. The length of the conductors within the interaction region is denoted as \(L\). The task is to calculate the magnetic force per unit length (\(F/L\)) between the two conductors.
For this problem, we will assume the currents are steady (DC), and neglect any edge effects by considering the conductors to be infinitely long. The expected result is a formula that relates the force per unit length to the currents in the conductors and their separation distance.
Hints
To approach this problem, recall Ampère's Law and how it relates the magnetic field around a long straight conductor to the current flowing through it. Remember that the force on a current-carrying conductor in a magnetic field is given by \(F = I(L \times B)\), where \(I\) is the current, \(L\) is the length vector of the conductor, and \(B\) is the magnetic field. Consider the direction of the magnetic field produced by each conductor and its effect on the other conductor. This understanding will guide you through the calculation process.
Solution
First, we use Ampère's Law to find the magnetic field (\(B\)) at a distance \(d\) from a long straight conductor carrying current \(I\). The law gives us \(B = \frac{\mu_0 I}{2\pi d}\), where \(\mu_0\) is the permeability of free space.
Next, calculate the magnetic field produced by the first conductor (\(I_1\)) at the location of the second conductor. According to Ampère's Law, this is given by \(B_1 = \frac{\mu_0 I_1}{2\pi d}\).
The formula for the force on a conductor in a magnetic field is \(F = I_2 L B_1\), where \(B_1\) is the magnetic field due to \(I_1\), and \(I_2\) is the current in the second conductor. Substituting the expression for \(B_1\) from the previous step into the force equation gives us \(F = I_2 L \left(\frac{\mu_0 I_1}{2\pi d}\right)\).
Simplifying the expression, we find the force per unit length (\(F/L\)) between the two conductors:
\[ F/L = \frac{\mu_0 I_1 I_2}{2\pi d} \]
This equation shows that the force per unit length depends inversely on the distance between the conductors and directly on the product of the currents.
The direction of the force can be determined using the right-hand rule, indicating that the force is attractive for parallel currents and repulsive for antiparallel currents.
Conclusion
The principle that the magnetic force per unit length between two parallel conductors depends inversely on their separation and directly on the product of the currents flowing through them has profound implications in electrical engineering and physics. This understanding is applied in the design of electromagnets, magnetic levitation systems, and is crucial for ensuring the stability of electrical power transmission lines.
The ability to harness and manipulate electromagnetic forces has paved the way for technological advancements that define our modern world. From the generation and distribution of electricity to the development of advanced transportation systems, the principles underlying the magnetic force between parallel conductors continue to fuel innovation and drive progress in science and engineering.
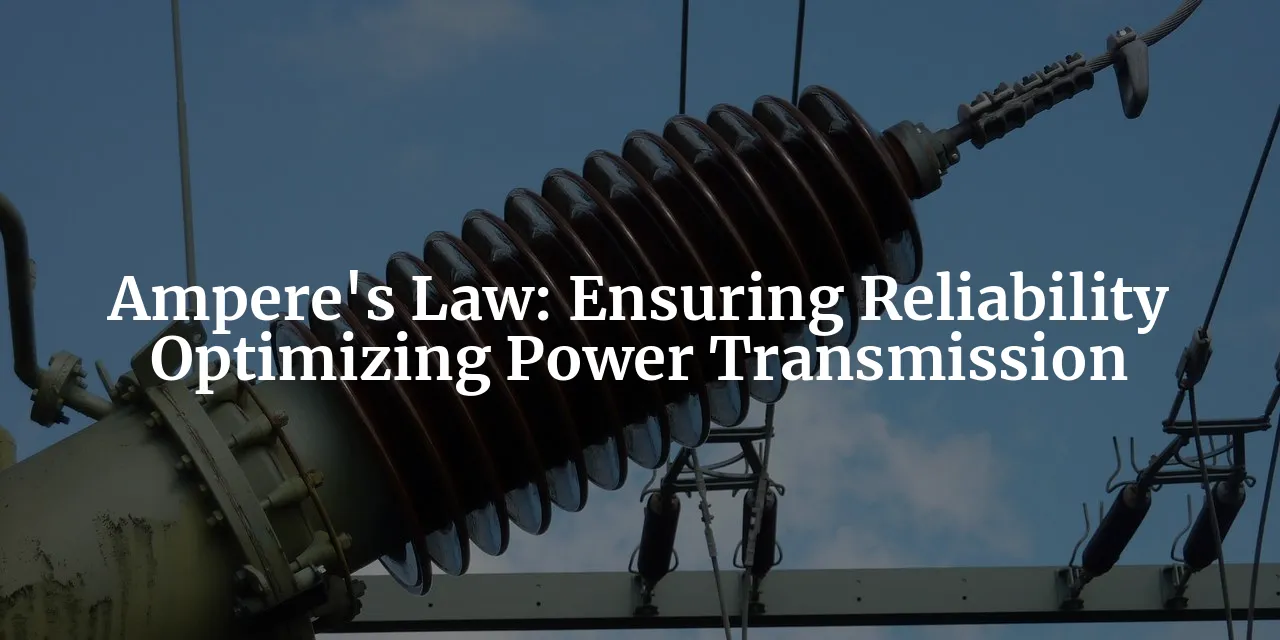
Background: Amperes Law: Preventing Sag in Overhead Power Lines
Overhead power lines are a cornerstone in the global transmission of electrical energy, enabling the flow of power across vast distances with remarkable efficiency. These lines, characterized by their suspended conductors supported by towers or poles, are pivotal in connecting power generation sites with consumers, thereby facilitating the demands of modern civilization. However, the reliability and efficiency of these transmission systems are contingent upon managing a seemingly mundane yet critically important phenomenon: the sagging of overhead conductors. Sag, or the vertical distance between the apex of a suspended conductor and its supports, is more than just a physical attribute; it is a critical factor that influences the tension and durability of power lines under various environmental conditions.
The significance of understanding and preventing excessive sag cannot be overstated, as it directly impacts the safety, cost, and performance of power transmission infrastructure. In this context, Ampere's Law, a fundamental principle in electromagnetism, plays a crucial role in comprehending the physics behind sag prevention, especially in the case of parallel conductors carrying electric currents. This law, which relates the integrated magnetic field around a closed loop to the electric current passing through the loop, offers insights into the forces at play in overhead power lines.
The evolution of high-voltage transmission lines, from the first practical use in telegraphy to the modern ultra-high voltage (UHV) lines, marks a significant advancement in our ability to transmit power over longer distances with minimal losses. This historical progression not only underscores the technological achievements in the field but also highlights the increasing complexity of managing the physical constraints of such infrastructure.
This article aims to provide undergraduate and graduate students of physics with a comprehensive exploration of the interplay between electrical principles and physical realities in the management of overhead power lines. By combining insights from physics, history, and engineering, we will delve into how theoretical concepts are applied to address practical challenges in power transmission, with a particular focus on preventing sag in overhead conductors. Through a detailed and scientifically accurate narrative, we endeavor to illuminate the fascinating world of power transmission and the pivotal role of Ampere's Law in ensuring the reliability and efficiency of this global infrastructure.
The Physics of Overhead Power Lines
At the heart of electricity transmission lies the interplay between voltage, current, and resistance—three fundamental concepts in the study of electricity ↗. High voltage transmission lines, capable of carrying voltages as high as 500 kV, exemplify the principle that for a given amount of energy, increasing the voltage reduces the current. This reduction in current is crucial for minimizing resistive losses and the heating of conductors, thereby enhancing the efficiency of power transmission over long distances.
Ampere's Law, integral to understanding the magnetic fields around conductors, reveals the magnetic interactions between parallel conductors carrying currents. According to this law, expressed mathematically as \(\oint \mathbf{B} \cdot d\mathbf{l} = \mu_{0}I\), where \(\mathbf{B}\) is the magnetic field, \(d\mathbf{l}\) is an element of length around the closed loop, \(\mu_{0}\) is the permeability of free space, and \(I\) is the current through the loop, the magnetic field around a conductor carrying current induces forces on nearby conductors carrying currents in parallel. These forces are central to understanding the behavior of suspended conductors in overhead power lines.
The shape of a conductor strung between two towers approximates that of a catenary. This geometric shape is not merely by design but is a result of the physical behavior of the conductor under the influence of gravity, where the sag of the conductor varies depending on temperature and additional loads such as ice cover. The catenary curve, therefore, provides a fundamental model for understanding the sag in overhead power lines.
Environmental factors like wind, sunlight, and ice significantly affect the sag and tension in overhead line conductors. For instance, ice accumulation increases the weight of the conductor, thereby increasing its sag, while wind can induce oscillations that further complicate the tension dynamics of the line. These factors necessitate a comprehensive approach to designing and maintaining overhead power lines to ensure their reliability and efficiency under varying environmental conditions.
To calculate the sag in overhead conductors, a simplified mathematical model can be employed, incorporating the physical properties of the conductors, the span between support towers, and environmental load factors. While Ampere's Law provides the foundation for understanding the magnetic interactions between parallel conductors, the calculation of sag involves considering the gravitational forces acting on the conductor, tension in the conductor, and the geometric properties of the catenary curve.
The physics of overhead power lines is a complex interplay of electrical principles and physical realities. From minimizing resistive losses through high voltage transmission to managing the sag and tension of conductors under environmental loads, the design and maintenance of these lines require a deep understanding of both electrical laws and physical models. Ampere's Law, in particular, offers critical insights into the magnetic interactions that influence the behavior of parallel conductors, underscoring the importance of electromagnetism in the field of power transmission.
Historical Developments in Power Transmission
The journey of power transmission through overhead lines is a testament to human ingenuity and the relentless pursuit of technological advancement. From the first practical application in telegraphy, the evolution of overhead power lines has been marked by significant milestones that have reshaped the landscape of electrical transmission. The inception of high-voltage transmission traces back to 1882, with a pioneering project between Munich and Miesbach, covering a distance of 60 km 1. This groundbreaking endeavor laid the foundation for the future of power transmission.
As the demand for electricity grew, so did the need for higher voltages and more efficient transmission methods. The invention of strain insulators emerged as a pivotal development, enabling the use of higher voltages in overhead lines. These insulators had to be robust enough to support the weight of the conductor spans, as well as withstand additional loads from ice accumulation and wind 1. The role of strain insulators cannot be overstated, as they significantly enhanced the reliability and safety of high-voltage transmission lines.
The progression towards ultra-high voltage (UHV) lines marked another leap forward in the capacity to transmit power over vast distances. UHV lines, characterized by their ability to operate at voltages exceeding 765,000 volts, have made it possible to transmit five times more power over six times the distance compared to conventional lines 1. This advancement not only optimized the efficiency of power transmission but also opened up new possibilities for a global electricity grid, as highlighted by The Financial Times calling UHV lines a "game changer" 1.
The introduction of composite core conductors, such as the ACCR and ACCC conductor, represented a significant innovation in reducing line losses and preventing sag. These conductors, with their reduced thermal sag, allowed for up to twice the current carrying capacity compared to traditional conductors. The ACCC conductor, in particular, with its carbon and glass fiber core, demonstrated a coefficient of thermal expansion about 1/10 that of steel. This innovation enabled the incorporation of 28% more aluminum without any diameter or weight penalty, thereby reducing line losses by 25 to 40% depending on the electric current 1.
The historical trajectory of overhead power lines is a narrative of overcoming challenges through innovation. From the first high-voltage transmission to the advent of UHV lines and the development of advanced conductors, each milestone has contributed to the efficient and reliable transmission of electrical energy across great distances.
Engineering Solutions to Minimize Sag
The engineering of overhead power lines involves a meticulous consideration of various factors to ensure efficiency, reliability, and safety. One of the paramount challenges in the design and construction of these lines is minimizing sag—the vertical distance between the lowest point of the conductor and the two support points of transmission towers. Sag not only influences the physical structure of the power lines but also affects their operational integrity and safety 2.
To address this challenge, engineers employ several strategies, starting with the selection of conductor material. The bare wire conductors, typically made of aluminum or steel, are chosen for their balance of strength, conductivity, and weight. The use of composite core conductors, like ACCR and ACCC, further exemplifies the engineering efforts to reduce thermal sag while enhancing current carrying capacity 1.
Bundle conductors represent another critical solution in minimizing sag and improving transmission efficiency. By consisting of several parallel cables connected at intervals by spacers, bundle conductors mitigate the corona effect and enhance cooling due to increased surface area. This configuration not only improves transmission efficiency but also reduces sag by distributing the load more evenly across multiple cables 1. Furthermore, the lower reactance and avoidance of ampacity reduction due to the skin effect underscore the multifaceted benefits of bundled conductors in addressing engineering challenges 1.
The role of insulators in overhead power lines is also crucial in minimizing sag. Developments in insulator technology, particularly the shift towards polymer insulators for UHV lines, have significantly impacted the reliability and performance of power transmission systems. Polymer insulators, with their hydrophobic characteristics, offer improved wet performance, reducing the risk of sag and enhancing the overall stability of the line 1.
Maintaining safe tension in conductors is another vital aspect of minimizing excessive sagging. Engineers must ensure that conductors are kept at a tension that prevents over sagging while avoiding undue pressure between supports. This balance is crucial for the structural integrity of the power lines and the prevention of power failures 2.
The engineering solutions to minimize sag in overhead power lines encompass a comprehensive approach that includes the careful selection of materials, the application of advanced technologies, and a deep understanding of the physical dynamics at play. Through these efforts, engineers continue to enhance the efficiency, reliability, and safety of power transmission systems, meeting the growing demands of our modern world.
The Role of Amperes Law in Preventing Sag
Amperes Law, a fundamental principle in electromagnetism, plays a pivotal role in the design and operation of overhead power lines. This law, formulated by André-Marie Ampère in the 1820s, relates the integrated magnetic field around a closed loop to the electric current passing through the loop. In the context of overhead power lines, Amperes Law can be applied to understand and optimize the magnetic interactions between parallel conductors carrying current.
The law is mathematically expressed as:
where \(\mathbf{B}\) is the magnetic field, \(d\mathbf{l}\) is an element of the loop, \(\mu_0\) is the permeability of free space, and \(I\) is the current through the wire. For parallel conductors, the magnetic field produced by one conductor exerts a force on the other, affecting its physical spacing and tension. This interaction is crucial in minimizing the sag of power lines.
By adjusting the current levels in these conductors, it is possible to manipulate the magnetic forces between them to counteract gravitational forces that cause sagging. Theoretically, an optimal current can be calculated that minimizes sag while ensuring efficient power transmission. This involves a delicate balance between the magnetic forces, which tend to pull the conductors together, and the mechanical tension that keeps them apart.
In practice, engineers use these principles to design power lines that are both stable and reliable. For example, increasing the current in parallel lines can reduce sag by strengthening the magnetic attraction between them. However, this must be done within the limits of thermal heating and other practical constraints.
The challenges of applying Amperes Law in real-world scenarios include the complexity of environmental conditions, such as wind and ice, which can significantly affect the sag and tension in power lines. Despite these challenges, ongoing research into electromagnetic principles and advanced materials offers potential breakthroughs in optimizing power transmission infrastructure. For instance, the use of composite core conductors with reduced thermal expansion coefficients allows for higher current carrying capacity with minimal sag.
Environmental and Safety Considerations
The construction and operation of overhead power lines have significant environmental and safety implications. One of the primary concerns is visual pollution and habitat disruption. Overhead lines, especially those that traverse wilderness areas or scenic landscapes, can detract from the natural beauty and potentially harm local ecosystems 1. To mitigate these impacts, engineers and planners carefully consider the routing of power lines and, in some cases, opt for undergrounding despite its higher costs.
Wildlife and aircraft collisions with power lines present another safety concern. Birds, in particular, are at risk of electrocution or collision with the wires. To minimize these risks, power lines near airfields are marked on maps and equipped with conspicuous plastic reflectors to enhance visibility 1. Additionally, modern designs include features like bird diverters that make the lines more visible to avian species.
The sagging and oscillation of conductors not only pose a risk of power outages but also increase the likelihood of faults due to contact with trees or other objects. Regulatory and industry standards dictate the construction and maintenance practices for overhead power lines to ensure they withstand environmental stresses and minimize safety hazards 3.
Moreover, the trade-offs between overhead and underground power lines are a subject of ongoing debate. While underground lines have less environmental impact and are less susceptible to weather-related disruptions, they are significantly more expensive to install and maintain. This cost difference often makes overhead lines the preferred choice for long-distance transmission 1.
Community engagement and public awareness are crucial in addressing concerns related to overhead power lines. By involving local communities in the planning process and educating the public about the safety measures in place, utilities can foster a better understanding of the necessity and benefits of these infrastructures.
The design and operation of overhead power lines involve a complex interplay of physical laws, engineering practices, and environmental and safety considerations. By leveraging the principles of Amperes Law and adhering to rigorous standards, it is possible to optimize the reliability and efficiency of power transmission while minimizing its impact on the environment and public safety.
Conclusion
The management of sag in overhead power lines is a multifaceted endeavor that requires a deep understanding of physics, engineering, and environmental considerations. The interplay between electrical principles and physical realities, as exemplified by the application of Ampere's Law, underscores the complexity of optimizing power transmission infrastructure for efficiency, reliability, and safety.
By delving into the physics of overhead power lines, we have explored the fundamental concepts of voltage, current, resistance, and magnetic interactions that underpin the design and operation of these transmission systems. The historical developments in power transmission, from the first high-voltage lines to the advent of ultra-high voltage (UHV) lines and advanced composite core conductors, have reshaped the landscape of electrical transmission, emphasizing the relentless pursuit of technological advancement in the field.
Engineering solutions to minimize sag, such as the selection of conductor materials, the use of bundle conductors, and advancements in insulator technology, highlight the meticulous considerations required to address the physical dynamics of power lines. The role of Amperes Law in preventing sag, by optimizing the magnetic interactions between parallel conductors, offers a theoretical framework for designing stable and reliable power transmission systems.
Environmental and safety considerations, including visual pollution, habitat disruption, wildlife and aircraft collisions, and the trade-offs between overhead and underground power lines, underscore the need for a comprehensive approach to balancing the demands of electrical energy with the preservation of the natural environment and public safety.
In light of the interdisciplinary nature of addressing sag in overhead power lines, it is essential to encourage students and researchers to explore further the fascinating intersection of physics, engineering, and environmental science in power transmission. Ongoing challenges and future directions in optimizing overhead power lines for efficiency, reliability, and minimal environmental impact call for continued innovation and collaboration across disciplines.
For further reading, we recommend exploring the works of pioneers in the field of power transmission, as well as recent research articles on the application of electromagnetism in the design of overhead power lines. By fostering a deeper understanding of the physical principles and practical challenges in power transmission, we can meet the growing global demand for electrical energy while ensuring the sustainability and resilience of our transmission infrastructure.
The study of Ampere's Law and its application in preventing sag in overhead power lines offers a rich and rewarding journey into the intersection of physics, engineering, and environmental science, with far-reaching implications for the future of electrical energy transmission.
References